Running the clock backward reveals spacetime “disintegrating” in the early universe
Perimeter postdoctoral researcher Barbara Šoda has been using spectral geometry to describe “fluctuating” spacetime.
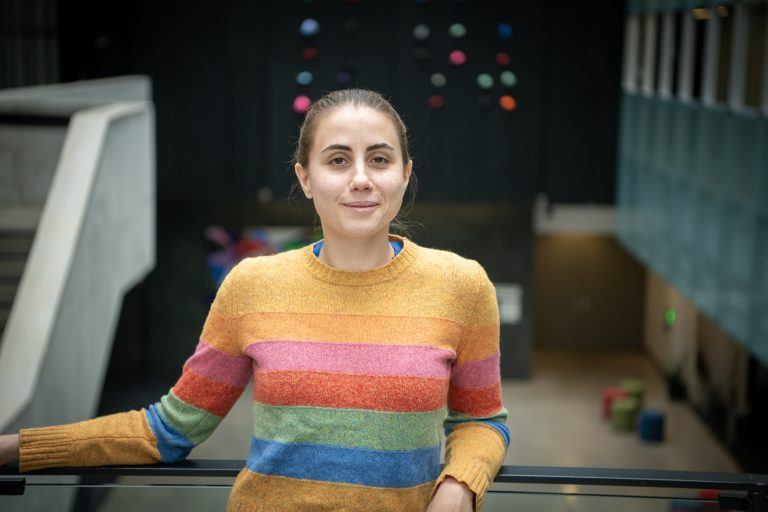
The early universe was a place of extremes, where tiny fluctuations could shape the very structure of the universe, and the laws of physics themselves. And all of it emerged, it seems, from a soup of formless quantum information.
“If we could journey back towards the big bang’s fiery temperatures, we might eventually find that spacetime disintegrates,” says Barbara Šoda, a postdoctoral researcher at Perimeter Institute.
She worked with Achim Kempf, a professor at the University of Waterloo and a Perimeter affiliate, and Marcus Reitz of Jagiellonian University in Poland on “Model for Emergence of Spacetime from Fluctuations,” recently published in Physical Review Letters.
The work suggests how spacetime could emerge from shapeless bits of quantum information and that spacetime could change its very structure and number of dimensions, such as going from two dimensions to the three dimensions of space (and one of time) we are familiar with.
Transitions at different temperatures – such as going from steam to water to ice – are common in nature. Spacetime, likewise, could have fluctuated and undergone such transitions.
The study shows how the transitions would happen because of two fundamental physics phenomena – fermionic push and bosonic pull.
Fermions are the foundational particles of all matter – these include quarks, electrons, neutrinos, protons, and neutrons. They are individualistic by nature – they want to push away from each, as described by the famous Pauli exclusion principle, which says identical fermions cannot occupy the same quantum state. The pressure of fermionic push is what keeps a neutron star from collapsing in on itself.
Bosons, on the other hand, are more like social groupies and can pile together in the same quantum state. Bosons pull together, and that is how strange states of matter like Bose-Einstein condensates are possible. Bosons carry energy and forces. A photon, for example, is a boson that carries the electromagnetic force. The gluon, Z boson, W boson, and Higgs boson are other examples.
The same sort of push and pull can happen to spacetime, causing it to fluctuate. “As the temperature is increased, the balance of the two phenomena can change, leading to a change in the dimensions of spacetime,” Šoda says. “If temperatures are extreme enough, this balance could be disrupted, leading to the disintegration of spacetime.”
This theory offers a fresh perspective on the universe’s origins, adds Šoda.
Kempf says the math used to develop this picture is especially interesting.
Šoda and her colleagues used mathematical tools of spectral geometry – that is all about relating the spectra of sounds to shapes – developed by Hermann Weyl more than 100 years ago in Germany.
One way to think about Weyl’s mathematics, Kempf says, is to ask: “If you clink a spoon against a vase, to what extent can we describe the curvature of a vase from just its sound? Weyl’s work provided a way to use information about spectra, the frequencies present in a sound, and relate those to shapes such as curvature.”
How does that relate to spacetime? Well, Einstein’s general relativity theory is all about the curvature of spacetime, he says. Moreover, “there are always quantum fluctuations, as if spacetime is ringing a little bit,” Kempf explains.
Imagine having an extremely high-resolution microscope to probe the structure of spacetime at very high energies. You would find that as you zoom into smaller and smaller distances, you would get spacetime fluctuations because of the uncertainty principle.
“In the very early universe, when everything was so hot and everything was happening at very, very, small distances, there were energy fluctuations that led to curvature fluctuations,” Kempf explains.
The result would be spacetime that bubbles into existence, like a piece of molten glass that bubbles into a bit of a curve, bursts, and disappears. “Spacetime could have these bubbles that burst, and at some point, if you reach far enough back to where the energy is too high and everything is too hot, the whole thing just disintegrates,” he says.
Weyl’s mathematics provides a more powerful and simpler way of describing these transitions in the spacetime manifold, Kempf adds. In trying to describe spacetime, mathematically, one can get stuck with four dimensions, or two dimensions, yet not know how to move from one to the other. But with spectra it is easier to do. “The mathematics of sound is much more flexible,” he says.
Šoda says the paper relates “species of particles” – the various bosons and the fermions – to the number of dimensions of spacetime. This is something that, to her knowledge, has not been done before.
“The fact that different species of particles come into play at different energies is well-known in standard particle physics,” Šoda says. In particle colliders such as at CERN, for example, the higher they can boost the energy levels, the more species of particles they find.
“In this paper, we found that we could connect the balance of the numbers of bosonic and fermionic species of particles to the number of dimensions,” Šoda says.
All of this plays into the grand quest of modern physics research, which is to unite our understanding of general relativity (Einstein’s gravity theory) with quantum theory, which describes the interactions of fundamental particles and forces in nature.
Trying to fit gravity and quantum theory together into one all-encompassing and coherent framework is not easy. Both theories are enormously successful in their own right, but they do not speak the same language and are mathematically incompatible with one another. By relating particles to dimensions of spacetime, the recent paper provides new insights.
There have been various approaches to arriving at a unified theory of quantum gravity – such as string theory and loop quantum gravity – but mathematically, this is a difficult nut to crack. The usual method is to “quantize” gravity, by considering it as being made up of tiny, discrete building blocks but somehow forming the continuous smooth manifold that we see in the “classical” Einstein gravity picture.
Šoda has also recently published a paper with Jonathan Oppenheim, a professor of quantum theory at University College London, who has developed yet another approach to resolving the conundrum.
Oppenheim is suggesting that if there are random fluctuations in spacetime and the gravitational field is jiggling around, one can arrive at a “hybrid” theory where the quantum particles and classical spacetime interact. In that case, spacetime would not need to be quantized to arrive at a consistent theory.
Oppenheim, Šoda, Carlo Sparaciari (a research fellow at University College London), and Zachary Weller-Davies (a recent Perimeter postdoctoral researcher who is now in London) devised a way of testing his theory. This is outlined in the paper, “Gravitationally induced decoherence vs space-time diffusion: testing the quantum nature of gravity,” published in Nature Communications in December 2023.
It posits that Oppenheim’s “postquantum theory of classical gravity,” which predicts random fluctuations in spacetime, can also render the apparent weight of objects unpredictable if the objects are measured precisely enough. The paper outlines how ultra-precise measurements of mass and interferometry experiments could be used to place some bounds on how much Einstein’s classical gravity theory can interact with quantum matter, to either prove or rule out the theory.
Oppenheim has made a bet with Geoff Penington of Cambridge University and Carlo Rovelli, an Italian physicist who is a Distinguished Visiting Research Chair at Perimeter, that gravity doesn’t need to be quantized to arrive at a coherent picture. The person (or persons) winning the 5,000-to-one bet can get 5,000 bazinga balls (or potato crisps or shots of olive oil, according to their fancy).
Kempf says Šoda is an expert at the mathematical calculations needed to work out these theories. She is pleased that the mathematical calculations she works on can deepen our understanding of how spacetime formed. “This is a step towards that,” she says of her recent research.